Cryogenic nitrogen in the food and beverage industry
The adoption of cryogenic processes in the food and beverage industry is predominantly driven by liquid nitrogen (LIN), and it is the preferred candidate for food chilling and freezing compared to mechanical refrigeration techniques. This summary document presents significant applications of cryogenic nitrogen along the food supply chain, including food freezing, food processing, product packaging, refrigerated storage, and transportation.
Properties of liquid nitrogen
The primary attributes of liquid nitrogen (LIN) that make it a preferred candidate in the Food and Beverage sector include its chemical inertness, high volume expansion ratio associated with liquid-to-vapor phase change, and the high enthalpy of vaporization. The chemical inertness and non-corrosive nature of nitrogen permit its contact with foods without adversely affecting organoleptic properties. Notably, the superior thermophysical properties of LIN enable its use as a refrigerating agent. The high volume expansion ratio of LIN makes it also suitable for certain inert packaging applications (i.e., LIN dosing).
When a liquid cryogen is injected into a target medium, it undergoes a phase change into saturated vapor, followed by a temperature increase of the vapor phase to ambient temperature. The ability of a liquid cryogen to remove heat from the target medium depends on the latent heat of vaporization for its liquid-to-saturated vapor phase change and the sensible heat difference associated with the temperature increase of the vapors. A LIN-based cooling process is associated with an enthalpy of vaporization of 199 kJ/kg[1] (at 1 bar, -196 °C). In addition, a sensible heat capacity of 1 kJ/kg/K of the generated N2 vapors permits an additional 216 KJ/kg with an assumed temperature gradient of 216 °K at room temperature (-196 °C to 20 °C). This corresponds to a net heat removal of almost 415 kJ/kg N2 from the target medium at room temperature. In addition to the enthalpy consideration, the heat transfer rate is also controlled by the thickness and thermal conductivity of the nitrogen vapor resulting from the Leidenfrost phenomenon.
Another major LIN characteristic with significant equipment design and safety implications is the volumetric expansion coefficient. The liquid-to-vapor phase transition of nitrogen is associated with a volumetric expansion ratio of 1:694[1] at 20 °C.
Benefits of liquid nitrogen for food applications
The adoption of cryogenic technologies like liquid nitrogen and liquid CO2 offers several benefits for food chilling and freezing, and refrigeration applications. These include higher throughput, enhanced product quality, and reduced product loss during processing.
Higher throughput and easier downstream processing
The high latent heat associated with nitrogen phase change enables an ultra-fast cooling of the entire volume of the product within just a few minutes of exposure to liquid nitrogen. This high cooling power allows fast, uniform, and efficient cooling of the products. For comparison, the heat transfer coefficient in a mechanical refrigeration-based blast freezer is around 17 W/m2/K (for air velocity of 2.5 m/s), increasing to 100-140 W/m2/K in the LIN spray zone of cryogenic tunnel freezer [2]. Compared to mechanical refrigeration, a higher temperature differential also exists between the cooling medium and the product to be frozen in cryogenic freezing. This enables faster freezing of the products in a cryogenic system.
Furthermore, the lowest temperature attainable in mechanical refrigeration is around -40 °C[3], whereas temperatures as low as -196 °C could be achieved in a direct-contact LIN-based cryo-system. The use of cryo-freezers also makes it possible to achieve Individual Quick Freezing (IQF) of products with no clumping of the products after freezing (i.e. free-flowing). This enables easier downstream processing and packaging of frozen products.
Enhanced product quality
One significant drawback of food freezing using mechanical refrigeration is the use of cold, dry air blasts for extended periods to reduce the internal product temperature of poultry, meat, and seafood to desired target levels. This process results in dehydration or loss of moisture from the exposed surfaces and adversely affects the texture and flavor of the cooked product due to reduced water content on the skin surface[4]. The quality of fresh-cut fruits and vegetables also suffers from slow freezing processes, as slow freezing regimes tend to form large and non-uniform ice crystals[5]. This often results in squishy fruits once they are thawed after frozen storage. The underlying reason is the formation of large ice crystals with sharp edges within the product during slow freezing that puncture the cell wall structure, leading to cellular damage upon thawing[5]. This is referred to as "drip losses". In some products, a shorter freezing time can significantly reduce drip loss. For example, in strawberries, drip loss decreases from 20 % for a product frozen in 12 hours to 8 % for a 15 min freezing time. Losses are further reduced to less than 5 % for an 8 min freezing time in a cryogenic freezer[6]. This makes liquid nitrogen a preferred option for food chilling and freezing.
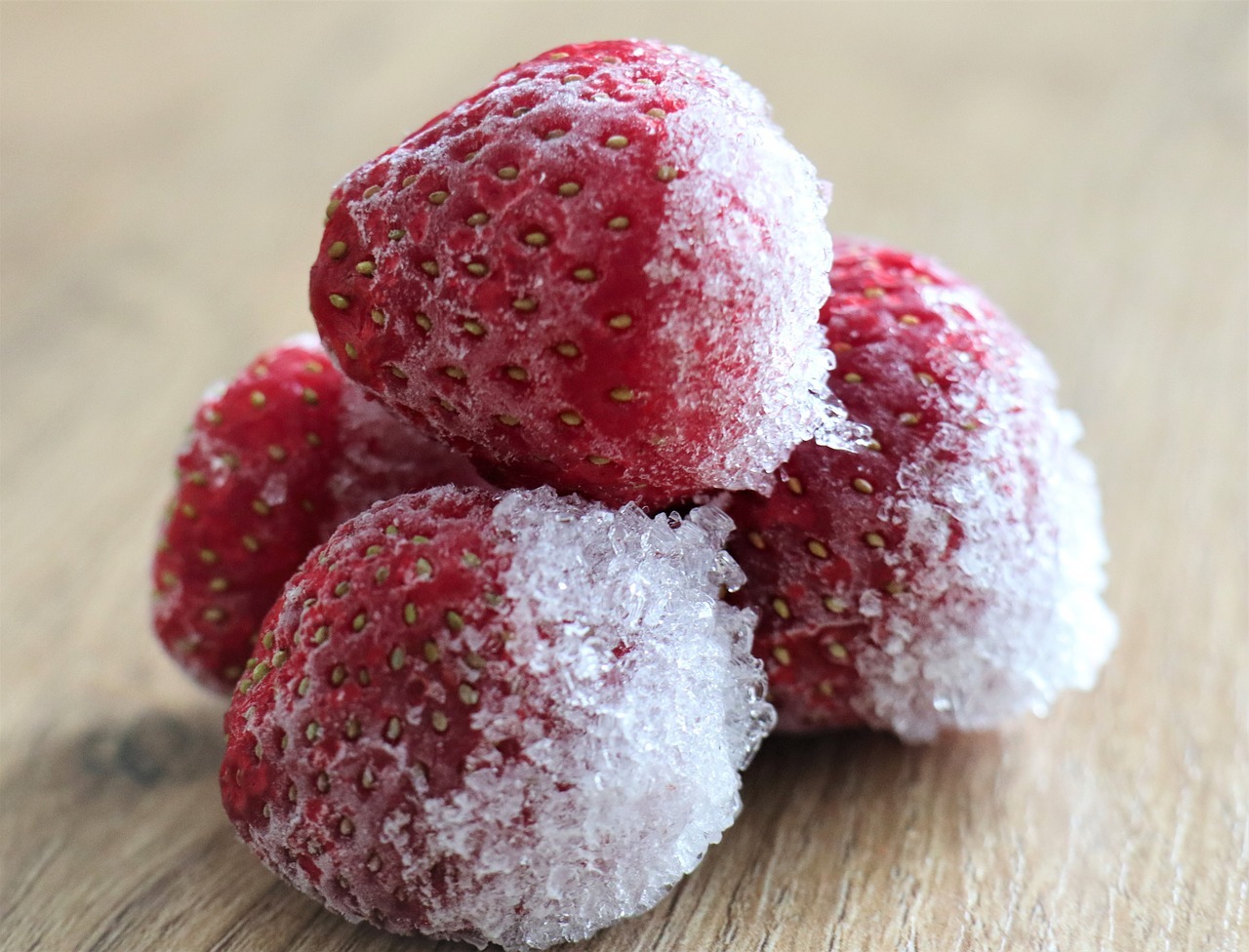
The dehydration and texture damage during freezing could be prevented by using ultra-fast freezing with LIN. The texture preservation can be attributed to the reduced osmotic imbalance within the cells[7] and the formation of microscopic ice crystals[5] within the product which are less harmful to the texture[8]. This helps preserve the product's shape even after thawing and prevents clumping. Furthermore, rapid freezing using LIN results in the formation of a solid ice crust over the surface that prevents moisture loss from the product. The same principle of rapid surface cooling using LIN is also employed for freezing ready-to-eat or catered foods like packaged meals, pizzas, pies, pastries, and cakes during transportation and storage, with the shape, texture, and mouthfeel preserved after thawing.
Another application of LIN in food freezing involves the application of a protective glazing layer of ice over the frozen surface (especially in fish fillets[9]). This prevents the dehydration and quality loss of the frozen products during storage[10]. The use of cryogenics also aids in reducing the proliferation of spoilage and pathogenic microorganisms on the product surface. The ultra-fast freezing achieved using LIN can lower the product temperature to a safe level within a short period, thus effectively minimizing the time the product remains at elevated temperatures conducive to microbial growth[2].
Environmental impact
In recent years, there has been significant concern about the high Global Warming Potential (GWP) of the refrigerant gases used in mechanical refrigeration. In the food industry, those typically include R134a, R404A, and R22, which have GWP values of 1430, 3922, and 1810 respectively [11], compared to a reference GWP value of 1 for CO2. With annual leak rates of these refrigerant gases in commercial refrigeration installations estimated to be between 11 % and 30 % [12], the use of LIN for food freezing and chilling applications offers a solution with higher sustainability. A study evaluating the environmental impact of cryogenic refrigerating systems for food transport reported that operation related GHG emissions (i.e. direct emissions) were next to negligible in these systems. However, production related emissions of cryogenic fluids (i.e. indirect emissions) should also be monitored when considering the environmental impact throughout the entire life cycle of the equipment[13].
Liquid nitrogen for food freezing
Maintenance of an optimal temperature level without significant fluctuations is an indispensable part of the food supply chain, which extends across various domains like food processing, product packaging, storage and transportation, and retail service[14]. In addition to preventing spoilage from bacterial and enzymatic activity, it is also essential for the adopted cooling technology to preserve the organoleptic quality and nutritional profile of fresh produce and processed foods.
Liquid nitrogen (LIN) cryogenic freezers
Various equipment is used in the food industry for LIN-based chilling and freezing applications depending on the throughput required, the desirable footprint of the unit, and targeted quality standards.
Cryogenic cabinets
Cryogenic cabinet freezers are utilized for batch-freezing applications and are equipped with multiple LIN injection nozzles and blower fans for uniform temperature distribution[15]. Industrial batch freezers use trays of product (fresh, raw, or fully cooked and ready to eat) loaded into an insulated chamber. LIN is then dosed directly into the chamber at controlled amounts, with the product cooling achieved by convection. The frozen products are removed from the chamber after the desired residence time.
Cryogenic freezer tunnels
Cryogenic freezer tunnels are utilized for continuous freezing and chilling applications. When utilized for product chilling, these units are beneficial for scenarios in which the product temperature needs to be lowered for downstream processes (such as dicing to reduce the amount of fines) or to meet regulatory requirements for hot foods in which the temperature reduction needs to be achieved within a specific time allowance to prevent pathogen growth. Cryogenic freezer tunnels employ moving conveyor belts with uniformly spaced nozzles that spray measured doses of LIN on the product to be frozen or chilled[16]. A top injection approach, in which LIN is sprayed over the food materials to be frozen/chilled without direct contact between the LIN nozzles and the product, is adopted in the freezer tunnel. LIN is sprayed as a fine mist on the product within the freezing zone. Internal fans are used for improved and uniform convection effects. Product cooling is mainly achieved by liquid-to-vapor phase change of LIN with additional heat removal from the product by convection effects from counter-current cold N2 gas[17]. The cryo-tunnel design guarantees a continuous and inline operation with minimal footprint and high-performance standards in terms of throughput and energy efficiency[16].
Cryogenic fluidized bed freezers
Fluidized bed freezers involve direct LIN spraying from the nozzles, as in the case of freezer tunnels, and employ an additional fluidized bed zone through high-speed convection fans. This increases the heat transfer rate, allowing the product to freeze quickly with minimal water loss. In addition, the residence time of foods within the freezer is significantly shortened - typically around 5-30 seconds, enabling high throughput and reduced equipment footprint. Cryogenic fluidized freezers are usually adopted for freezing small, uniform-sized foods like peas or sliced carrots, which are well-dewatered.[6]
Cryogenic spiral freezers
Cryogenic spiral freezers are used for the cryo-freezing of large quantities of products. The design of these units involves upward or downward spiral conveyor belts transporting the products through a cooling compartment. Multiple belt entrance or exit configurations could be implemented in these units, which improves the versatility of the production lines in handling various product loads and achieving the final product temperatures. LIN is continuously sprayed into the compartment through the nozzles incorporated into the outer walls of the cooling chamber, with convection fans for uniform distribution of the cold N2 gas throughout the compartment. The convection effects from cold N2 gas play a dominant role in product cooling. The significant benefits of a spiral freezer design are its lower footprint compared to a tunnel freezer for the same throughput and high utilization efficiency of the cryogen to achieve a temperature reduction of the product to the desired level[18]. However, it has a slower freezing rate than a tunnel freezer design.
Cryogenic immersion freezers
Immersion freezers involve immersing the product in a shallow trough of LIN, with the product transported through the trough onto the exit conveyor using a continuous current of LIN[19] or by introducing an external vibratory motion in the LIN trough[20]. The highest freezing rates attainable with LIN immersion enable its adoption for deep-freezing and crust-freezing of sticky and hard-to-freeze products, with several benefits like preserving product texture and minimal moisture loss during freezing[19]. The ability to achieve crust-freezing using LIN immersion enables its adoption as a pre-treatment step for sticky products like marinated poultry or seafood, with immersion freezing preventing the product from sticking to the conveyor belts in a downstream tunnel freezer unit[19]. The major disadvantage of the immersion freezer design is its high consumption of LIN when utilized for deep freezing (3-4 kg N2/kg product in an immersion freezer vs. 1 kg N2/kg product in a tunnel freezer[17]). The cryogen utilization efficiency in a processing plant utilizing an immersion freezer could be enhanced by using the cold nitrogen vapors from the immersion freezer exhaust in a downstream cryogenic freezer (tunnel freezer/spiral freezer).
Limitations of liquid nitrogen (LIN) in food freezing
Although cryogenic refrigeration offers many benefits, like lower initial capital investment and maintenance costs, it is associated with higher operating costs due to the consumption of LIN as the direct cooling medium. An operating cost comparison of cryogenic freezing vs. mechanical freezing is complicated by the geographical variations and seasonal volatility in the electricity prices, with an increase in electricity costs having a more significant impact on the economics of the mechanical freezing process compared to cryogenic freezing. In a comparative study with an electricity cost of 0.047 €/kWh and LIN cost of 0.08 €/kg, a higher cost of 0.07 €/kg product was reported for cryo-freezing of minced beef compared to 0.01 €/kg product for mechanical freezing[17]. The overall cost of processing however favored cryo-technology in this evaluation due to improved quality (0.14 €/kg product for cryo-freezing vs. 0.28 €/kg product for mechanical freezing[17]). Currently, the LIN freezing process is limited to high-value products like processed poultry/meat products, seafood, and easily perishable fruits (strawberries, raspberries, etc.), where the quality enhancement and value addition with LIN usage could offset the additional costs of LIN consumption.
Although LIN has been generally accepted in the food and beverage industry to better preserve the quality of frozen food, some studies have indicated a deterioration in quality with LIN freezing compared to conventional freezing techniques, especially cracking of products at high freezing rates. The outer layer is initially frozen during the food freezing process, with the freezing front moving gradually into the product interior. While the water-to-ice phase change is associated with a volumetric expansion, cooling of the frozen layer is associated with volumetric contraction. This results in outward tensile stress (on the frozen outer layer) and inward compressive stress (on the inner layers undergoing freezing). In the cryogenic freezing process, the high freezing rates do not allow enough time for stress dissipation within the product, which could sometimes form cracks in the frozen product[21]. Investigation performed on a lab-scale cryogenic system consistently observed cracks in products with lower porosity and higher density (e.g., margarine and butter). In contrast, no cracks during cryo-freezing were observed in products with higher porosities and lower density (e.g., french bread, pound cake)[22]. This was attributed to better stress dissipation in products with increased porosity, as well as lower density resulting from a lower moisture content in foods[22].
Finally, the adoption of cryogenic technologies requires uninterrupted LIN supply for food processing installations[23]. Depending on their LIN consumption rate, food processors most often rely on on-site bulk storage tanks filled using LIN trailer deliveries. Thus, access to a reliable supply of food grade LIN conforming to applicable regulatory and industry standards (e.g. ISO 22000 and FSSC 22000) is a prerequisite for adopting LIN-based cryo technologies in the food and beverage industry.
Liquid nitrogen for inert packaging
The high volumetric expansion ratio and chemical inertness of LIN extend its usage for non-carbonated beverage packaging applications. Once bottles are filled with the product, it is necessary to preserve their structural integrity during storage and transportation. This is challenging in the case of PET bottles, which are widely preferred due to their light weight, but are prone to collapse under weight (e.g., when stacked). Dosing the bottles with controlled amounts of LIN pressurizes these bottles due to the high volumetric expansion of LIN. Individual cryogenic nozzles are utilized in inert packaging applications that demand an accurate LIN dosing. While preserving the stability of these bottles[24], LIN dosing also creates an inert oxygen-deficient environment inside the package, protecting its content from oxidation or microbial spoilage from aerobic microorganisms[24].
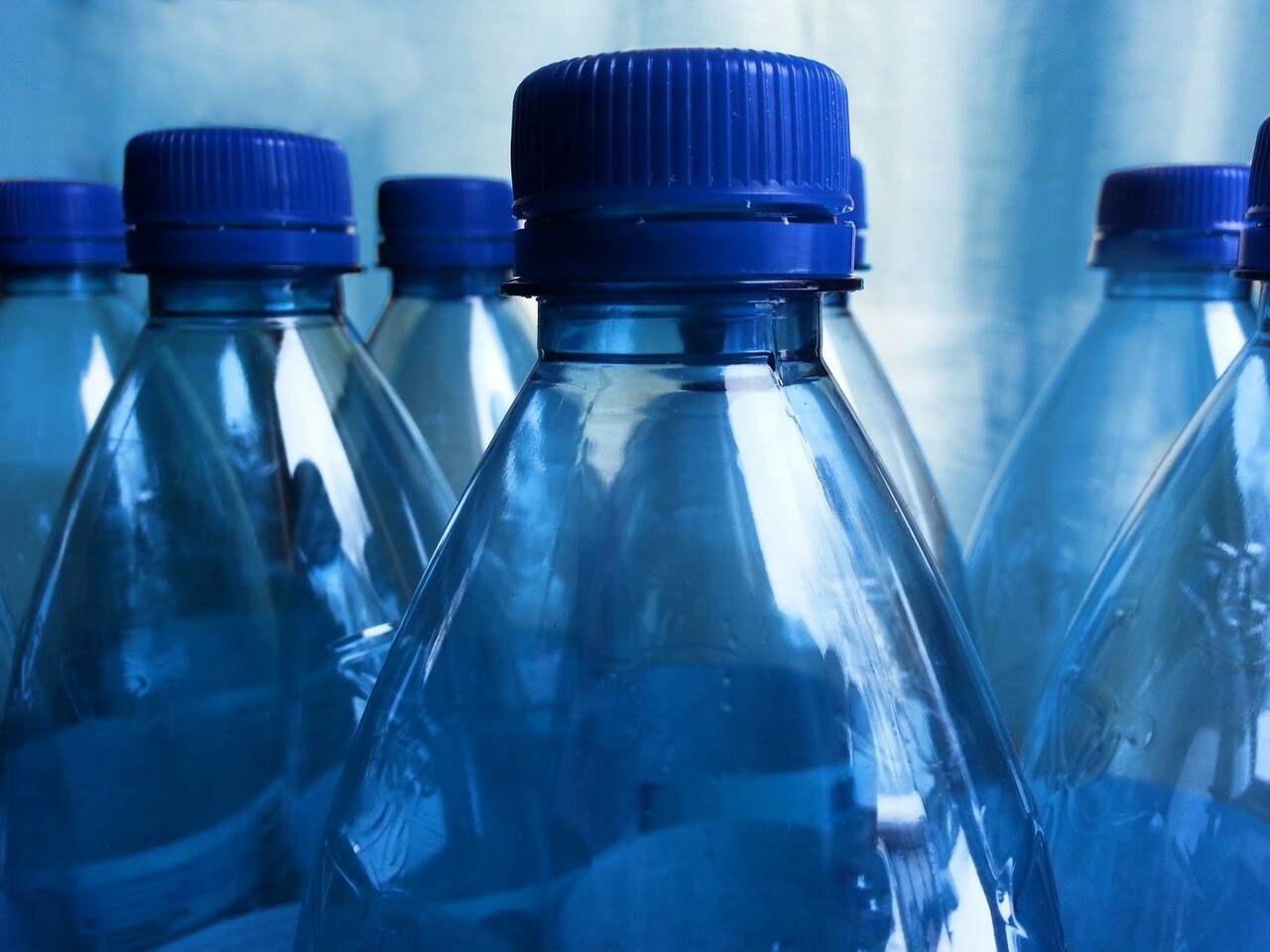
Liquid nitrogen for food processing
Liquid nitrogen (LIN) for food grinding
Spice grinding processes involve significant heat generation through friction. This results in a loss of volatile compounds from the product, which are essential for the flavor and aroma of the spices. Cryo-grinding technology in which LIN is injected at controlled rates into the grinding process offers a route to minimize this loss of volatile compounds / essential oils from the ground product. This is achieved through the removal of accumulated heat during the grinding process. In addition, direct contact with LIN makes the material brittle and easier to grind, thereby reducing operating costs and particle size distribution[25]. The utilization of LIN to maintain temperature during grinding is also adopted in meat mixing processes, in which elevated temperatures might increase the risk of microbial growth.
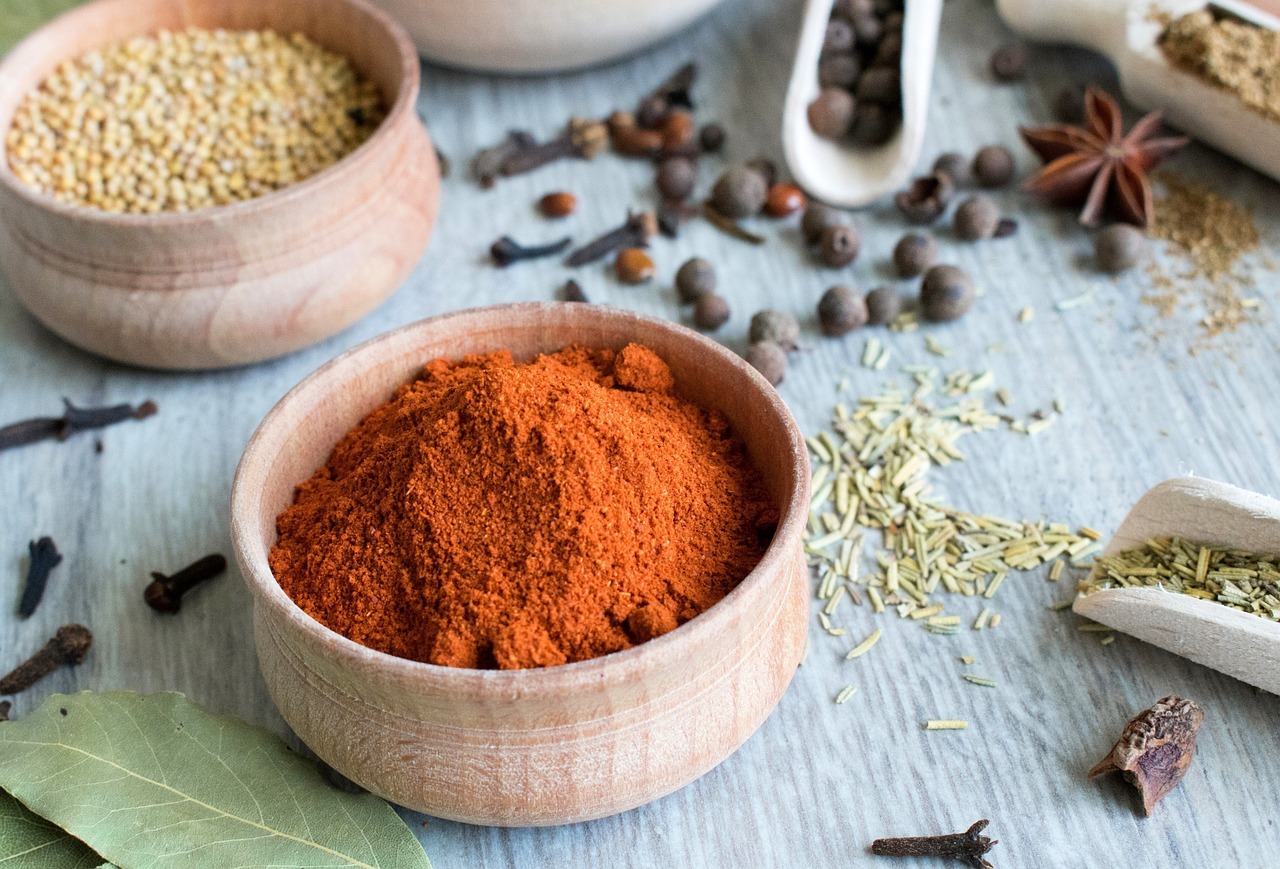
Most of the cryogenic food grinding and mixing processes adopt a bottom-injection method for LIN injection, in which LIN is combined with the food materials through a nozzle in contact with the product. In this approach, the generated cold N2 gas comes into contact with the product, and the sensible heat transfer significantly contributes towards the overall cooling effect[17], enabling a higher cryogen utilization efficiency. However, some bottom injection nozzle designs can be prone to clogging and microbial contamination due to insufficient sanitation. Also, some processors prefer adopting a top-injection approach in certain applications to eliminate direct contact between LIN nozzles and the product.
Liquid nitrogen (LIN) for cryogenic spray chilling and encapsulation
The production of value-added food ingredients benefits significantly from adopting cryogenic technologies. For example, spray chilling in a LIN-based atmosphere is adopted to freeze and pelletize perishable foods from their liquid phase[26]. The process referred to as "cryo-crystallization" could also be utilized for the crystallization of high melting point liquids (like fats, oils etc) to obtain desired crystal structures for confectionary fats and bakery shortenings[17]. In this process, the solid feed is initially heated to liquefy it, and then sprayed through a nozzle as a fine spray. LIN is injected beneath the nozzle, and feed liquid is exposed to a co-current stream of liquid and vaporized N2. In an alternate design, liquefied feed could be injected through perforated plate with nozzles, with LIN injected into a separate chamber and the cold N2 gas entering into the feed liquid chamber in counter current direction. This allows the vertical flow path of individual dropping liquid droplets to be maintained undisturbed, thus preventing the coalescence of drops and yielding a product with highly monodispersed beads for use as food supplements[27].
The spray-chilling technique utilizing liquid nitrogen is also beneficial for encapsulating bio-active food ingredients[28] (like nutrients, preservatives, antioxidants, etc.) in a matrix (comprising starch or lipids)[29]. Microencapsulation technologies offer several benefits, such as improved stability, enhanced bio-availability, and targeted release of the bio-active compounds[30].
Liquid nitrogen (LIN) for specialty foods
Ultra-fast freezing using LIN is utilized in the food industry to produce confectionaries and specialty foods with complex geometric patterns, which are not otherwise possible using conventional freezing approaches[31]. The technique is also utilized in making ice cream bars with multiple layers, with each layer frozen with liquid nitrogen before applying the following layer[17]. For the production of ice creams, the use of LIN for freezing yields a smooth and creamy texture due to the small crystal size of water and fat crystals[32] and reduced crystal aggregation[33].
Liquid nitrogen (LIN) for food ingredient testing
Differential Scanning Calorimetry (DSC) techniques adopted for ingredient testing and phase evaluation in food processing operations relies on cryo-stabilization using liquid nitrogen[34]. DSC testing plays an instrumental role in maintaining optimal conditions for the processing, storage, and transportation of foods and various plant/animal derivatives through a characterization of the glass transition temperature of its ingredients.
Liquid nitrogen for refrigerated storage and transportation
There is an increased focus on adopting LIN for cold chain applications, including refrigerated storage and temperature-controlled transportation, which is currently dominated by vapor compression cycles relying on liquid refrigerants. Refrigerated transportation trucks are designed with multi-temperature compartments, which enables simultaneous transportation of foods with varying temperature and humidity requirements (e.g., 13-18 °C for bananas, 0-1 °C for beef, -3-1 °C for eggs).[35]
The use of LIN for refrigerated storage/transportation applications utilizes cryogenic storage tanks that hold the cryogen and are attached to the refrigerated compartment. Cryogenic cooling is then achieved in two ways. In the first approach, controlled amounts of LIN are injected directly into the refrigerated space using nozzles to regulate the temperature levels. This approach is, however, of limited application due to the safety and health concerns of an anoxic environment. An alternate method that is being commercialized involves an indirect LIN usage as a refrigerant in a heat exchanger, with the cold air circulated continuously through the refrigerated compartments [36].
Cryogenically cooled trucks offer several benefits over vapor compression-cycle based refrigerated transportation that relies on fossil fuels (diesel). The refrigerants utilized in the vapor compression cycle (like HFCs) have a significant Global Warming Potential (GWP) and may have flammability concerns[37]. Field trials have also demonstrated enhanced performance and reduced operating costs with LIN-cooled truck refrigeration compared to a diesel-powered cooling unit[36].
Useful links for further information
Zhao, Y., Ning, J., & Sun, Z. (2022). Study on liquid nitrogen and carbon dioxide combined jet quick-frozen strawberry. International Journal of Refrigeration, 136, 1-7. https://iifiir.org/en/fridoc/study-on-liquid-nitrogen-and-carbon-dioxide-combined-jet-quick-frozen-145063
Yang, S., Hu, Y., Takaki, K., Yu, H., & Yuan, C. (2021). Effect of water ice-glazing on the quality of frozen swimming crab (Portunus trituberculatus) by liquid nitrogen spray freezing during frozen storage. International Journal of Refrigeration, 131, 1010–1015. https://iifiir.org/en/fridoc/effect-of-water-ice-glazing-on-the-quality-of-frozen-swimming-crab-144594
Cryogenic solutions: innovations to serve refrigerated transportation for sustainable and efficient cold chain. 5th IIR International Conference on Sustainability and the Cold Chain. Proceedings: Beijing, Chine, 6-8 avril 2018. https://iifiir.org/en/fridoc/cryogenic-solutions-innovations-to-serve-refrigerated-transportation-33435
Pathier D., Gerard A., Denavaut M. Ultra fast freezing of warm product using cryogenic slush. 1st IIR International Conference of Cryogenics and Refrigeration Technology (ICCRT 2016). Proceedings: Bucharest, Romania, June 22-25, 2016. https://iifiir.org/en/fridoc/ultra-fast-freezing-of-warm-product-using-cryogenic-slush-32078
Kumar, A., Chandra, B. H., Sunil, S., & Kulkarni, G. V. (2021). Application of cryogenics in grinding of spices for value addition: A Review. NVEO- Nat. Volatiles & Essent. Oils, 2021; 8(5):10580-10593. https://www.nveo.org/index.php/journal/article/view/2982
Rai, A., & Tassou, S. A. (2017). Energy demand and environmental impacts of alternative food transport refrigeration systems. Energy Procedia, 123, 113-120. https://doi.org/10.1016/j.egypro.2017.07.267
Gibbs, W. W., & Myhrvold, N. (2011). Cryogenic Cooking. Scientific American, 305(2). https://doi.org/10.1038/scientificamerican0811-31
Acknowledgements
This summary document was prepared by Midhun Joy (Senior Research Scientist - R&D Life Sciences Group, Air Liquide) and Srinivas Vanapalli (University of Twente, member of the IIR A2 commission on “Gas liquefaction and separation”). The authors would like to express their gratitude to Bill Adams (Director - Food and Pharmaceutical Solutions, Airgas) for his input on industrial cryogenic systems. The document was designed and translated into French by Monique Baha (IIR Scientific and Technical Information Department). It was proofread by Nathalie de Grissac, under the supervision of Jean-Luc Dupont (Head of the Scientific and Technical Information Department).
References
1. Gas Encyclopedia by Air Liquide. (2023). Nitrogen. https://encyclopedia.airliquide.com/nitrogen#properties
2. George, R. M. (1993). Freezing proceseses used in the food industry. Trends in Food Science & Technology, 4(5), 134–138. https://doi.org/10.1016/0924-2244(93)90032-6
3. Le-Bail, A., & Jha, P. K. (2019). SubChapter 7.1.3. Application—Freezing of Foods. [Chapter 7.1—Refrigeration]. In R. Cachon, P. Girardon, & A. Voilley (Eds.), Gases in Agro-Food Processes (pp. 241–252). Academic Press. https://doi.org/10.1016/B978-0-12-812465-9.00014-1
4. James, S. J., & James, C. (2002). Meat refrigeration. Woodhead Publishing Ltd. https://www.sciencedirect.com/book/9781855734425/meat-refrigeration
5. Nesvadba, P. (2008). Thermal Properties and Ice Crystal Development in Frozen Foods. In Frozen Food Science and Technology (pp. 1–25). John Wiley & Sons, Ltd. https://doi.org/10.1002/9781444302325.ch1
6. ASHRAE. (2018). Chapter 29. Industrial food freezing systems. In 2018 ASHRAE Handbook. Refrigeration. SI edition. https://iifiir.org/en/fridoc/2018-ashrae-handbook-refrigeration-si-edition-4791
7. Berk, Z. (2018). Food process engineering and technology. Academic press. https://www.sciencedirect.com/book/9780123736604/food-process-engineering-and-technology
8. Sanz, P. D., de Elvira, C., Martino, M., Zaritzky, N., Otero, L., & Carrasco, J. A. (1999). Freezing rate simulation as an aid to reducing crystallization damage in foods. Meat Science, 52(3), 275–278. https://doi.org/10.1016/S0309-1740(99)00002-9
9. Yang, S., Hu, Y., Takaki, K., Yu, H., & Yuan, C. (2021). Effect of water ice-glazing on the quality of frozen swimming crab (Portunus trituberculatus) by liquid nitrogen spray freezing during frozen storage. International Journal of Refrigeration, 131, 1010–1015. https://doi.org/10.1016/j.ijrefrig.2021.06.035
10. Vanhaecke, L., Verbeke, W., & De Brabander, H. F. (2010). Glazing of frozen fish: Analytical and economic challenges. Analytica Chimica Acta, 672(1), 40–44. https://doi.org/10.1016/j.aca.2010.03.045
11. Devecioğlu, A. G., & Oruç, V. (2015). Characteristics of Some New Generation Refrigerants with Low GWP. Energy Procedia, 75, 1452–1457. https://doi.org/10.1016/j.egypro.2015.07.258
12. Francis, C., Maidment, G., & Davies, G. (2017). An investigation of refrigerant leakage in commercial refrigeration. International Journal of Refrigeration, 74, 12–21. https://doi.org/10.1016/j.ijrefrig.2016.10.009
13. Rai, A., & Tassou, S. A. (2017). Energy demand and environmental impacts of alternative food transport refrigeration systems. Energy Procedia, 123, 113–120. https://doi.org/10.1016/j.egypro.2017.07.267
14. Kitinoja, L. (2013). Use of cold chains for reducing food losses in developing countries (No. 13–03; PEF White Paper, Issues 13–03). The Postharvest Education Foundation (PEF). http://postharvest.org/Cold_chains_PEF_White_Paper_13_03.pdf
15. Air Liquide. Cryo cabinet. Retrieved 28 April 2023, from https://uk.airliquide.com/equipment/food-industry-equipment/cryo-cabinet
16. Air Liquide. Cryo Tunnel ZR2. Retrieved 28 April 2023, from https://uk.airliquide.com/equipment/cryo-tunnel-zr2
17. Girardon, P., Moziar, C., & Pathier, D. (2019). SubChapter 7.1.4.1. Cryogenics for Food Freezing, Chilling, and Temperature Control Applications. [Chapter 7.1—Refrigeration]. In R. Cachon, P. Girardon, & A. Voilley (Eds.), Gases in Agro-Food Processes (pp. 241–252). Academic Press. https://doi.org/10.1016/B978-0-12-812465-9.00014-1
18. DSI Dantech. Cryogenic spiral freezers. Retrieved 28 April 2023, from https://dsidantech.com/products/spiral-freezer-cryogenic
19. Air Liquide. Cryo immersion unit ACC. Retrieved 28 April 2023, from https://za.airliquide.com/cryo-immersion-unit-acc
20. Air Liquide. Cryo Immersion Unit CFV. Retrieved 28 April 2023, from https://za.airliquide.com/cryo-immersion-unit-cfv
21. X. Shi, A. K. D., S. Mukherjee. (1999). Thermal Fracture in a Biomaterial During Rapid Freezing. Journal of Thermal Stresses, 22(3), 275–292. https://doi.org/10.1080/014957399280878
22. Kim, N.-K., & Hung, Y.-C. (1994). Freeze-cracking in Foods as Affected by Physical Properties. Journal of Food Science, 59(3), 669–674. https://doi.org/10.1111/j.1365-2621.1994.tb05590.x
23. ISO Standards. (2022, June 7). ISO 22000. Food safety management. ISO. https://www.iso.org/iso-22000-food-safety-management.html
24. Air Liquide. Carbonation. Retrieved 28 April 2023, from https://uk.airliquide.com/solutions/carbonation
25. Singh, K. K., & Goswami, T. K. (1999). Design of a cryogenic grinding system for spices. Journal of Food Engineering, 39(4), 359–368. https://doi.org/10.1016/S0260-8774(98)00172-1
26. Oxley, J. D. (2012). 5—Spray cooling and spray chilling for food ingredient and nutraceutical encapsulation. In N. Garti & D. J. McClements (Eds.), Encapsulation Technologies and Delivery Systems for Food Ingredients and Nutraceuticals (pp. 110–130). Woodhead Publishing. https://doi.org/10.1533/9780857095909.2.110
27. Air Liquide. NexeliaTM for Ingredient Processing. Retrieved 28 April 2023, from https://cn.airliquide.com/sites/al_cn/files/2022-08/3-nexelia_fp_pi_ingredients_processing_offer-sheet_en_lr_0.pdf
28. Đorđević, V., Balanč, B., Belščak-Cvitanović, A., Lević, S., Trifković, K., Kalušević, A., Kostić, I., Komes, D., Bugarski, B., & Nedović, V. (2015). Trends in Encapsulation Technologies for Delivery of Food Bioactive Compounds. Food Engineering Reviews, 7(4), 452–490. https://doi.org/10.1007/s12393-014-9106-7
29. F. Gibbs, S. K., Inteaz Alli, Catherine N. Mulligan, Bernard. (1999). Encapsulation in the food industry: A review. International Journal of Food Sciences and Nutrition, 50(3), 213–224. https://doi.org/10.1080/096374899101256
30. Anabio Technologies. Microencapsulation of Sensitive Ingredients. https://www.anabio.ie/encapsulation-benefits/why-micro-encapsulation/
31. Gibbs, W. W., & Myhrvold, N. (2011). Cryogenic Cooking. Scientific American, 305(2). https://doi.org/10.1038/scientificamerican0811-31
32. Petzold, G., & Aguilera, J. M. (2009). Ice Morphology: Fundamentals and Technological Applications in Foods. Food Biophysics, 4(4), 378–396. https://doi.org/10.1007/s11483-009-9136-5
33. Russell, A. B., Cheney, P. E., & Wantling, S. D. (1999). Influence of freezing conditions on ice crystallisation in ice cream. Journal of Food Engineering, 39(2), 179–191. https://doi.org/10.1016/S0260-8774(98)00161-7
34. Levine, H., & Slade, L. (1990). Cryostabilization technology: Thermoanalytical evaluation of food ingredients and systems. Thermal Analysis of Foods, 221–305. https://www.researchgate.net/publication/301701383_Cryostabilization_Technology_Thermoanalytical_Evaluation_of_Food_Ingredients_and_Systems
35. Brecht, J. K., Sargent, S. A., Brecht, P. E., Saenz, J., & Rodowick, L. (2019). Protecting Perishable Foods During Transport by Truck and Rail: HS1328, 4/2019. In USDA AMS Handbook vol. 669 (University of Florida, Institute of Food and Agricultural Sciences). http://www.dx.doi.org/10.9752/TS230.04-2019
36. Special Report: Cryogenic truck refrigeration with nitrogen. (2011, February). Global Cold Chain News. https://www.coldchainnews.com/Global_Special_Report-Cryogenics.pdf
37. McLinden, M. O., Seeton, C. J., & Pearson, A. (2020). New refrigerants and system configurations for vapor-compression refrigeration. Science, 370(6518), 791–796. https://doi.org/10.1126/science.abe3692
Search in the encyclopedia for articles on the following topics:
Cryogenics and gas processing | Refrigerants and secondary refrigerants | Refrigerating equipment | Cryobiology and cryomedicine, freeze-drying | Application of refrigeration to perishable products | Refrigerated storage | Heat pumps, energy recovery | General information on refrigeration, energy and environment