Cryogenics in the pharmaceutical industry: drug design and bioavailability improvement
Research activities in the pharmaceutical sector focus primarily on developing novel drug formulations which can elicit the desired response upon being administered to the patient with minimal side effects. Recent advancements in the cryogenic sector utilizing liquified-gas based cryogens offer significant potential to achieve this objective. In addition to their vital role in the development and optimal storage of Active Pharmaceutical Ingredients (APIs), these cryogenic processes are also capable of enhancing the bioavailability of drug formulations. This helps ensure a higher efficacy of medicinal interventions for the target diseases with minimal side effects. This summary document presents the potential applications of liquid cryogens in the pharmaceutical sector.
Cryogenic technologies based on liquefied gas
The commercial deployment of cryogenic solutions has expanded significantly in recent decades, from their early adoption to maintain the integrity of the cold chain to their use to induce changes in the physicochemical properties of materials. Compared to mechanical cooling-based cryogenic technologies that rely on vapor-compression cycles, the use of liquid cryogens (such as liquid nitrogen, helium, argon) offers the benefits of higher cooling rates, lower attainable temperature levels, uniform temperature distribution, absence of mechanical components, and reduced maintenance requirements. Various technologies have been developed in recent decades exploiting the potential of these liquid cryogens, namely cryogenic freezers that can guarantee uniform and fast cooling rates, cryo-based spray freezing and micronization processes that improve the bio-assimilation of pharmaceutical drugs, as well as novel routes for the production of active pharmaceutical ingredients.
Cryogenics thus offer immense potential to address a major challenge in the pharmaceutical industry, namely to develop drug formulations that can induce the desired therapeutic benefits with a minimal dosage of the medication to minimize side effects.
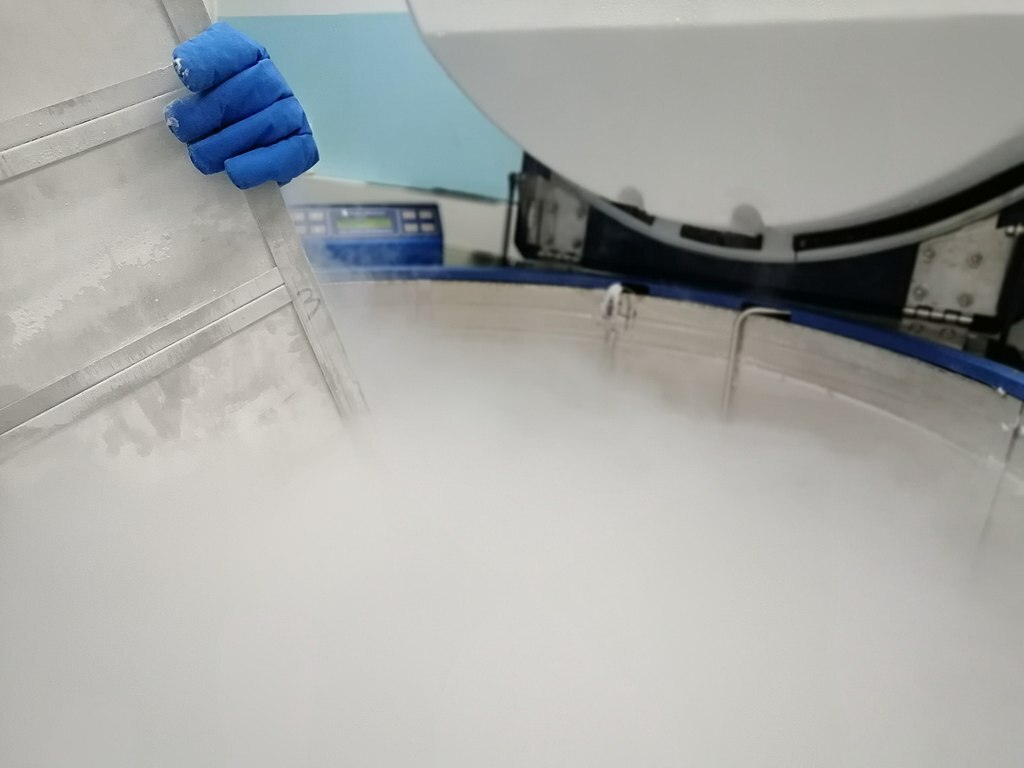
Cryogenics for improved bioavailability of orally administered drugs
Cryogenics-based technologies can play an important role in enhancing the ‘bioavailability’ of some orally-administered medications. Bioavailability refers to the fraction of the administered drug that reaches the blood circulation and its desired site of action in an active form [1]. It is controlled by both the ability of the drug to dissolve in the biological fluid around the mucous membrane (“aqueous solubility”) and its ability to penetrate through the mucous membrane (“Intestinal permeability”). Most of the drug formulations require a minimum effective concentration (MEC) threshold at the site of action to produce the desired pharmacological activity. Hence, for drugs with a low bioavailability, a high dosage needs to be administered to achieve MEC, which carries the risk of unintended adverse reactions.
The use of cryogenic technologies has been demonstrated to be effective in improving aqueous solubility, which in turn enhances dissolution in the biological mucous fluid in the digestive system. This has great potential in the pharmaceutical sector where almost 40% of the approved drugs are poorly soluble in water [2]. Research on the use of cryogenics-based technologies for improved bioavailability has focused on the development of nanostructured aggregates with increased surface areas [3] as well as converting the drug into an amorphous solid dispersion that has higher solubility than its crystalline counterpart [4].
Spray freeze-drying processes for enhanced solubility
The two major cryogenic processes currently being adopted for enhancing aqueous solubility include spray freeze drying (SFD) and spray-freezing into liquid (SFL) [5]. In these approaches, the solution containing the active ingredient of the drug along with the excipients and solvents is brought into contact with a cryogen like liquid nitrogen [3]. This causes a rapid freezing of the solution, after which the solvent is removed from the frozen solution using lyophilization or freeze-drying. The sublimation of the solvent yields dry powder comprising the active ingredient and excipients.
In the SFD process, an atomized spray of the drug solution is introduced into a cryogenic vapor zone. In contrast, in the SFL process commercialized by Dow Chemical Company, the atomized drug solution is sprayed directly into liquid nitrogen through nozzles immersed in the cryogen. The direct impingement of the drug solution into the liquid cryogen creates a higher degree of atomization compared to the SFD process, and eliminates the drawback of potential protein aggregation arising from gas-liquid interface in the SFD process [3].
The high degree of supercooling in the cryogenic process significantly limits the formation of crystal nuclei in the solution, along with reduced time available for crystal growth [6]. This induces the formation of amorphous particles in the solution instead of crystals that are normally formed under conditions of low degree of supercooling. The amorphous structure of the resulting powder, along with a significant increase in surface area [3] play a dominant role in improving the aqueous solubility of the active ingredients in the gastrointestinal system.
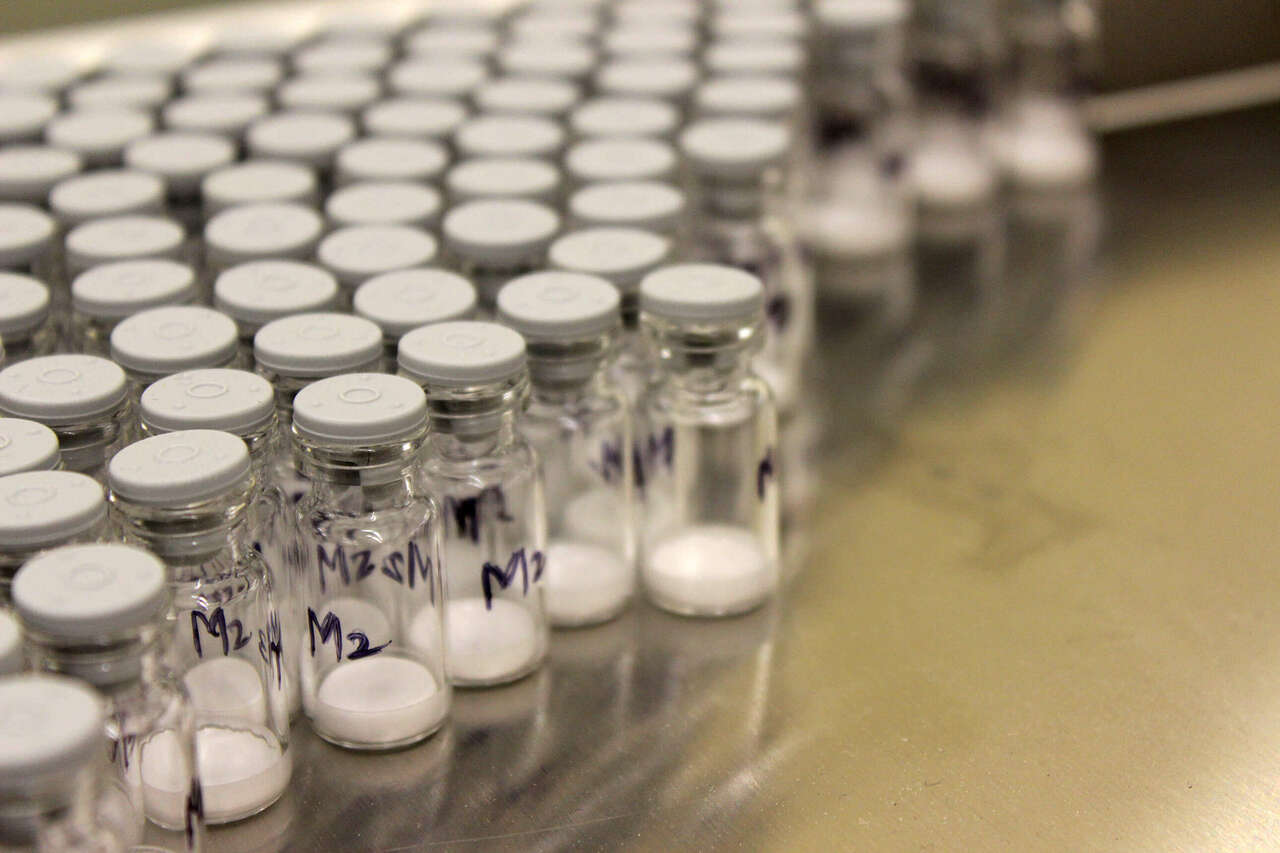
Micronization using cryogenic fluids
Micronization technologies, focused on reducing the particle size of API powders to micrometer levels in order to improve their solubility, could also benefit from cryogenics. The use of an ultra-low temperature environment offers a superior alternative to conventional spray-drying and milling techniques to reduce particle size of thermally or physically unstable APIs [7]. This could be implemented through a spraying of the solution directly into liquid nitrogen, with micronization achieved through liquid-liquid impingement [8].
Cryogenics for novel active pharmaceutical ingredients (API)
Cryogenic technologies also offer significant potential for the production and storage of active pharmaceutical ingredients (APIs), the class of compounds responsible for the therapeutic properties of drugs. Maintaining the desired stability or viability of these APIs is critical for generating the desired pharmacological response upon administration [9]. While these APIs can either be inorganic compounds (metal-ligand complexes, inorganic salts) [10] or protein-based organic compounds, optimal storage conditions are of paramount importance in the case of protein-based APIs. This is owing to the protein molecules being highly susceptible to denaturation and aggregation during the manufacturing processes resulting in loss of activity.
Cryofreezers to minimize denaturation in API compounds
The adoption of cryogenic freezers can ensure uniform cooling of the volume along with cooling rates fast enough to minimize the loss of protein activity [11] during the production, storage and transportation of APIs, thereby maintaining their stability and viability.
The necessity of such an optimal cooling technology arises from the dominant role of water molecules in maintaining the stability of the protein molecules, and its effects on intracellular and intercellular hydration [12]. A low cooling rate typically encountered in conventional freezers causes temperature non-uniformities in the solution, which causes non-uniform formation of ice and localized spots of high solute concentration. This results in aggregation and denaturation of the protein molecules. Also, an increased loss of water from the protein-ligand complex results in dehydration, causing irreversible damage to their structure and functionality [12]. The multiple drawbacks thus associated with conventional freezers could be overcome with the use of cryogenic technologies.
Cryotechnologies for production of novel API compounds
Cryogenic technologies also offer significant potential in the production and processing of novel APIs. Cryogenic conditions can induce molecular transformations that are otherwise impossible under normal process conditions [13].
This is especially true in the case of Grignard reactions where the Grignard’s reagent (organo-metallic reagent) reacts with aldehydes, ketones and esters to form new active ingredients [14]. The high-reactivity of the organometallic reagents makes their commercialization and scale-up challenging, with cryogen-modified conditions offering a potential alternative for performing controlled reactions [15]. A cryogenic reaction environment also offers the benefits of improved selectivity and elimination of undesired side-products in the synthesis process [16].
Cryo-imaging tools for drug design and development
The development of cryo-electron microscopy (Cryo-EM) overcomes the inherent challenge associated with the destruction of biological molecules due to high-energy electron beams involved in conventional electron microscopy techniques.
In cryo-EM techniques, the sample grid is immersed in liquid ethane or propane maintained at -190°C using liquid nitrogen [17]. This approach has the potential to play a dominant role in drug discovery, owing to the ability to image molecular structures to up to 1.25 Angstrom resolution [18]. This allows an accurate elucidation of protein structure and functionality [19], as well as binding modes and underlying mechanism of action of pharmaceutical drugs at the target site [20]. In addition, these imaging techniques are capable of generating high resolution images of pathogenic virus surfaces [21], thereby enabling researchers to identify possible target sites for drug candidates.
Useful links for further information
Adali, M. B., Barresi, A. A., Boccardo, G., & Pisano, R. (2020). Spray freeze-drying as a solution to continuous manufacturing of pharmaceutical products in bulk. Processes, 8(6), 709. https://iifiir.org/en/fridoc/spray-freeze-drying-as-a-solution-to-continuous-manufacturing-of-142837
Puri, M., Morar-Mitrica, S., Crotts, G., & Nesta, D. (2015). Evaluating Freeze–Thaw Processes in Biopharmaceutical Development. BioProcess International, 13(1), 34-45. https://bioprocessintl.com/manufacturing/fill-finish/evaluating-freeze-thaw-processes-biopharmaceutical-development-small-scale-study-designs/
Savjani, K. T., Gajjar, A. K., & Savjani, J. K. (2012). Drug solubility: importance and enhancement techniques. International Scholarly Research Notices, 2012. https://doi.org/10.5402/2012/195727
Willems, D., Benschop, T. Compact cryocooler developments. European Cryogenics Course 2022. https://iifiir.org/en/fridoc/compact-cryocooler-developments-145967
Acknowledgements
This summary document was prepared by Midhun Joy (Senior Research Scientist - Life Sciences Group, Air Liquide) and Srinivas Vanapalli (University of Twente, member of the A2 commission on “Gas liquefaction and separation”). It was designed and translated into French by Monique Baha (IIR head office). It was proofread by Philippe Lebrun (honorary member of the A1 commission on “Cryophysics, cryoengineering”) and Nathalie de Grissac, under the supervision of Jean-Luc Dupont (Head of the Scientific and Technical Information Department).
References
[1] Koch-Weser, J. Bioavailability of Drugs. N Engl J Med 291, 233–237 (1974). https://doi.org/10.1056/nejm197408012910505
[2] Rogers, T. L. et al. A novel particle engineering technology to enhance dissolution of poorly water soluble drugs: spray-freezing into liquid. European Journal of Pharmaceutics and Biopharmaceutics 54, 271–280 (2002). https://doi.org/10.1016/S0939-6411(02)00063-2
[3] Kalepu, S. & Nekkanti, V. Insoluble drug delivery strategies: review of recent advances and business prospects. Acta Pharmaceutica Sinica B 5, 442–453 (2015). https://doi.org/10.1016/j.apsb.2015.07.003
[4] Yang, W., Owens, D. E. & Williams, R. O. Pharmaceutical Cryogenic Technologies. in Formulating Poorly Water Soluble Drugs (eds. Williams III, R. O., Watts, A. B. & Miller, D. A.) vol. 3 443–500 (Springer New York, 2012). https://doi.org/10.1007/978-1-4614-1144-4_11
[5] Gurunath, S., Pradeep Kumar, S., Basavaraj, N. K. & Patil, P. A. Amorphous solid dispersion method for improving oral bioavailability of poorly water-soluble drugs. Journal of Pharmacy Research 6, 476–480 (2013). https://doi.org/10.1016/j.jopr.2013.04.008
[6] Overhoff, K. A. et al. Novel ultra-rapid freezing particle engineering process for enhancement of dissolution rates of poorly water-soluble drugs. European Journal of Pharmaceutics and Biopharmaceutics 65, 57–67 (2007). https://doi.org/10.1016/j.ejpb.2006.07.012
[7] https://www.fda.gov/media/75201/download.
[8] https://www.chemcon.com/fileadmin/chemcon.de/04_Downloads/ChemCon_Inorganic_APIs.pdf.
[9] https://bioprocessintl.com/manufacturing/monoclonal-antibodies/use-of-blast-freezers-in-vaccine-manufacture-322317/#CIT0006.
[10] Maurer, M. & Oostenbrink, C. Water in protein hydration and ligand recognition. J Mol Recognit 32, (2019). https://doi.org/10.1002/jmr.2810
[11] https://cdmo.seqens.com/api-manufacturing/cryogenics-how-cryogenic-capabilities-aid-api-development/.
[12] Wu, G. & Huang, M. Organolithium Reagents in Pharmaceutical Asymmetric Processes. Chem. Rev. 106, 2596–2616 (2006). https://doi.org/10.1021/cr040694k
[13] Pedersen, M. J. et al. Optimization of Grignard Addition to Esters: Kinetic and Mechanistic Study of Model Phthalide Using Flow Chemistry. Ind. Eng. Chem. Res. 57, 4859–4866 (2018). https://doi.org/10.1021/acs.iecr.8b00564
[14] https://cdmo.seqens.com/api-manufacturing/the-economics-of-cryogenic-chemistry-in-api-development/.
[15] Rogers, T. L., Johnston, K. P. & Williams, R. O. Solution-Based Particle Formation of Pharmaceutical Powders by Supercritical or Compressed Fluid CO2 and Cryogenic Spray-Freezing Technologies. Drug Development and Industrial Pharmacy 27, 1003–1015 (2001). https://doi.org/10.1081/DDC-100108363
[16] Gombotz, W. Process for producing small particles of biologically active molecules. https://patents.google.com/patent/US6569458B1/en
[17] Dubochet, J. & McDowall, A. W. Vitrification of pure water for electron microscopy. Journal of Microscopy 124, 3–4 (1981). https://doi.org/10.1111/j.1365-2818.1981.tb02483.x
[18] Yip, K. M., Fischer, N., Paknia, E., Chari, A. & Stark, H. Breaking the next Cryo-EM resolution barrier–Atomic resolution determination of proteins!. BioRxiv, 2020-05 (2020) https://doi.org/10.1101/2020.05.21.106740
[19] Nakane, T., Kotecha, A., Sente, A. et al. Single-particle cryo-EM at atomic resolution. Nature 587, 152–156 (2020). https://doi.org/10.1038/s41586-020-2829-0
[20] Van Drie, J. H. & Tong, L. Cryo-EM as a powerful tool for drug discovery. Bioorganic & Medicinal Chemistry Letters 30, 127524 (2020). https://doi.org/10.1016/j.bmcl.2020.127524
[21] Leigh, K. E. & Modis, Y. Imaging and visualizing SARS-CoV-2 in a new era for structural biology. Interface Focus. 11, 20210019 (2021). https://doi.org/10.1098/rsfs.2021.0019
Search in the encyclopedia for articles on the following topics:
Cryogenics and gas processing | Refrigerants and secondary refrigerants | Refrigerating equipment | Cryobiology and cryomedicine, freeze-drying | Application of refrigeration to perishable products | Refrigerated storage | Heat pumps, energy recovery | General information on refrigeration, energy and environment